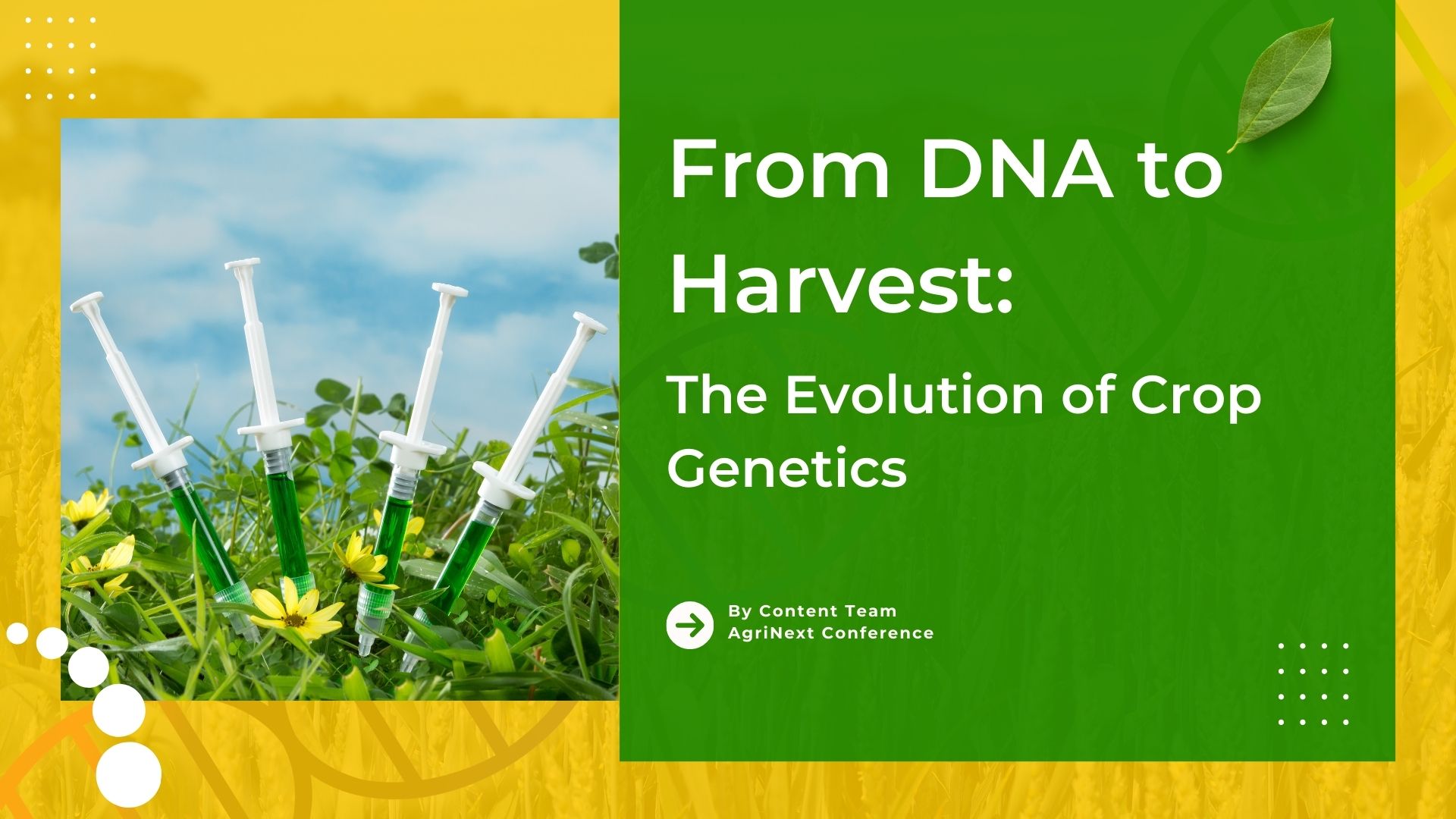
Introduction
Agriculture has always been at the heart of human civilization, but the way we grow food is changing rapidly. At the forefront of this transformation is the integration of advanced genetic techniques with innovative farming methods.
The evolution of crop genetics, from the understanding of DNA to modern agricultural practices, is a fascinating journey that encompasses several key stages. This evolution of crops has profoundly impacted the efficiency, yield, and resilience of crops, ensuring food security and sustainability. Here’s an overview of this evolution:
Table of Contents
Discovery of DNA and Genetic Principles
1866: Gregor Mendel’s experiments with pea plants laid the foundation of genetics by demonstrating inheritance patterns.
1953: James Watson and Francis Crick’s discovery of the DNA double helix structure provided insights into the molecular basis of heredity.
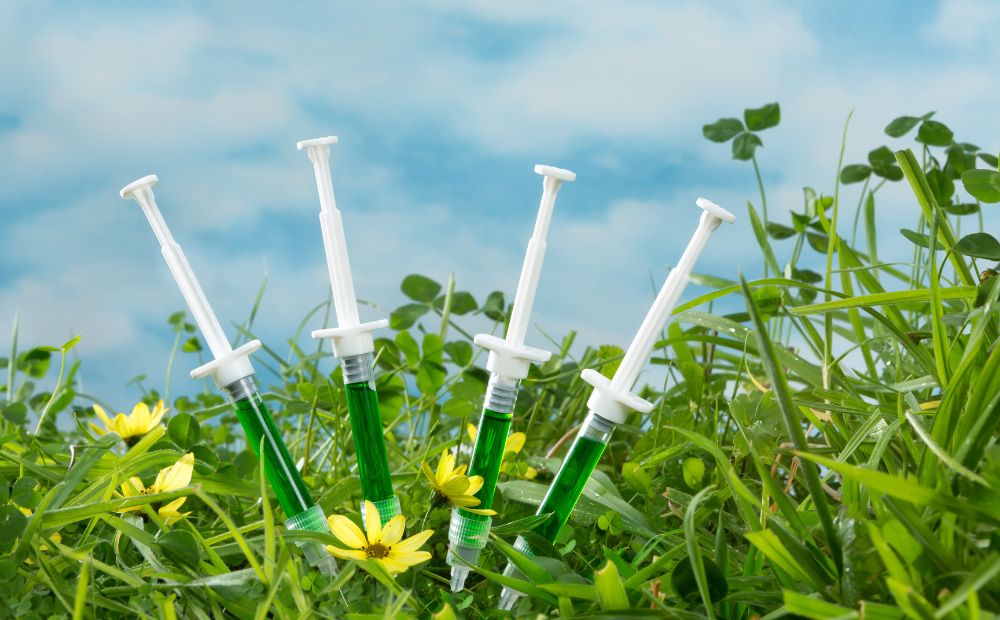
Crop genetics is transforming agriculture by unlocking the potential of plant DNA. This scientific approach enables the development of crops with superior traits such as enhanced yield, improved nutritional content, and increased resistance to pests and diseases. By reducing the reliance on chemical pesticides and fertilizers, crop genetics promotes more sustainable farming practices. Advanced techniques like CRISPR and marker-assisted selection allow for precise genetic improvements, accelerating the breeding process and helping crops adapt to climate change. The benefits of crop genetics are profound, ensuring a stable and secure food supply for a growing global population while promoting environmental sustainability.
Read more
Traditional Breeding Techniques
Selective Breeding:
Farmers and breeders select plants with desirable traits (e.g., size, taste, yield) and cross-breed them over multiple generations to enhance these traits.
Hybridization:
Crossing different plant varieties to produce hybrids with superior characteristics, such as disease resistance and higher yields.
Molecular Biology and Biotechnology
1970s-1980s: Development of molecular biology techniques, such as DNA sequencing and recombinant DNA technology, allowed scientists to understand and manipulate the genetic makeup of organisms.
Genetic Markers: Identifying specific sequences in DNA that are associated with desirable traits to assist in selective breeding (marker-assisted selection).
Genetically Modified Organisms (GMOs)
1990s: Introduction of genetically modified crops, where specific genes are inserted into plants to confer traits like pest resistance (e.g., Bt crops) and herbicide tolerance (e.g., Roundup Ready crops).
Regulation and Acceptance: GMOs are subjected to stringent regulatory scrutiny to ensure safety for consumption and environmental impact.
Genome Editing Technologies
CRISPR-Cas9: This groundbreaking technology allows precise editing of DNA, enabling the addition, removal, or alteration of specific genes in crops with high accuracy and efficiency.
Gene Drives: A method to ensure that a specific gene is inherited at a higher-than-normal rate, potentially useful for spreading beneficial traits rapidly in crop populations.
Genomics and Big Data
Whole-Genome Sequencing:
Sequencing the entire genome of crop species provides comprehensive insights into genetic variation and trait association.
Bioinformatics:
Leveraging computational tools to analyze large datasets, facilitating the identification of genetic markers and the understanding of complex traits controlled by multiple genes.
Synthetic Biology and Future Prospects
Synthetic Biology:
Designing and constructing new biological parts, devices, and systems, or redesigning existing biological systems for useful purposes in agriculture.
Sustainable Agriculture:
Developing crops that require fewer inputs (water, fertilizers, pesticides), are more resilient to climate change, and can improve nutritional content.
Key Aspects and Considerations
Food Security: Enhanced crop yields and resilience contribute to stable food supplies.
Environmental Sustainability: Reduced need for chemical inputs and improved resistance to biotic and abiotic stresses.
Ethical and Societal Issues: GMOs and gene-edited crops raise ethical questions and public concerns regarding safety, environmental impact, and labelling.
Genomics: A Potential Solution to the Perennial Problem
DNA genetic tools can significantly enhance breeding of perennial crops by identifying DNA variants linked to important traits, thereby accelerating the development of improved cultivars. This reduces the time and cost associated with traditional breeding methods, making it easier to introduce new, resilient varieties. By leveraging genetic diversity more effectively, these tools can help prevent perennial crops from falling prey to pests and diseases, ensuring sustainable food production.
Case Study:The Evolution of Maize:
Maize, one of the world’s most important crops, provides a prime example of crop evolution through domestication and genetic improvement. Originating from teosinte, a wild grass native to the Balsas River Valley in Mexico, maize underwent significant changes to become the staple crop we know today.
Key Milestones in Maize Evolution:
Domestication: Archaeological evidence suggests that maize was domesticated around 9,000 years ago by indigenous peoples in southern Mexico.
Genetic Studies: Modern genetic studies have traced the domestication process, identifying specific genes involved in the transformation of teosinte’s small, hard kernels into the large, soft kernels of maize.
Centers of Domestication and the Origin of Crops
Our review is limited to seed-propagated, annual crop species because they are the best-studied examples of domestication.
Historical Hypotheses on Domestication
The study of crop domestication origins was first scientifically pursued by Russian plant geneticist Nikolai Vavilov. In 1926, he proposed seven primary geographic “centers of domestication” based on regions with high crop varietal diversity. These centers are:China South Asia Southwest Asia (the Fertile Crescent)The Mediterranean Ethiopia Greater Mexico The Andes
Relevance to Maize:
Greater Mexico: Vavilov identified Greater Mexico as one of the primary centers of domestication, which aligns with the archaeological and genetic evidence of maize’s origins in this region. This supports the idea that regions with high diversity are key to understanding the domestication processes.
Hybridization and Increased Yields
By the early 20th century, scientists and farmers began to use hybridization techniques to improve corn yields. Hybrid corn, created by crossing different inbred lines, demonstrated hybrid vigour (heterosis), resulting in plants that grew faster and produced more kernels. This innovation led to the widespread adoption of hybrid corn in agriculture.
Molecular Biology Breakthroughs:
The discovery of the DNA double helix in 1953 by Watson and Crick revolutionized biology. In the 1970s and 1980s, advancements in molecular biology techniques, such as restriction enzymes and recombinant DNA technology, allowed scientists to manipulate the genetic material of organisms, including corn
The Rise of Genetically Modified Corn:
In the 1990s, genetically modified (GM) corn was introduced. Scientists inserted specific genes into corn plants to confer desirable traits. One of the first GM corn varieties was Bt corn, which contains a gene from the bacterium Bacillus thuringiensis. This gene produces a protein toxic to certain pests, reducing the need for chemical insecticides
Precision Breeding with CRISPR:
The advent of CRISPR-Cas9 in the 2010s marked a new era in crop genetics. This precise genome-editing tool allowed scientists to make targeted changes to the DNA of corn plants. For example, researchers have used CRISPR to enhance drought resistance in corn, which is crucial as climate change poses new challenges for agriculture.
Genomics and Big Data Integration
With the sequencing of the corn genome, scientists gained a comprehensive understanding of its genetic blueprint. This wealth of data, combined with advanced bioinformatics tools, enables the identification of genetic markers associated with important traits. Marker-assisted selection accelerates the breeding process by allowing breeders to select plants with desirable genes more efficiently.
Synthetic Biology and Future Prospects
Looking forward, synthetic biology offers exciting possibilities for corn cultivation. Scientists are exploring ways to engineer corn plants with new metabolic pathways to improve nutrient uptake, reduce fertilizer dependency, and even produce biofuels more efficiently.
The journey from the discovery of DNA to the modern genetic enhancement of corn exemplifies the remarkable progress in crop genetics. From traditional selective breeding to cutting-edge genome editing, each step has contributed to creating more productive, resilient, and sustainable corn varieties
Examples of Startups and Companies Involved in Maize Genetics
Here are a few examples of startups and companies involved in maize genetics:
Benson Hill and Brown Seed Genetics (Crop Genetics)
This company uses machine learning and big data analytics to accelerate plant breeding. They focus on improving crop traits, including those in maize, to enhance yield, nutritional content, and resilience.
Corteva Agriscience
As an agricultural division of DowDuPont, Corteva focuses on using advanced genetic technologies to develop superior maize hybrids. They invest heavily in biotechnology and genome editing tools like CRISPR.
Inari Agriculture
This startup leverages CRISPR and other gene-editing technologies to develop custom seed solutions. Their work includes optimizing maize genetics to increase productivity and sustainability.
Yield10 Bioscience :
This company works on improving crop yields through advanced metabolic engineering. They apply their technology to a variety of crops, including maize, to enhance productivity and environmental sustainability.
Syngenta India
Syngenta produces agrochemicals and seeds, it uses advanced genetic techniques to improve maize varieties. They focus on traits like pest resistance, herbicide tolerance, and increased yield.
These companies are at the forefront of integrating advanced genetics into maize cultivation, driving innovation in agriculture.
Conclusion
The evolution from basic understanding of DNA to advanced genetic manipulation techniques has revolutionized crop genetics. This progression not only addresses current agricultural challenges but also opens up new possibilities for future innovations in crop production and sustainability. The continuous advancement in genomics, biotechnology, and synthetic biology promises to further enhance the capabilities and resilience of crops, ensuring a secure and sustainable food supply for the growing global population.
Signup For AgriNext Conference Newsletter